Thus, the already aligned nuclei (parallel or anti-parallel to the applied steady external magnetic field B0), has now been perturbed owing to the ‘knocking’ by the ‘magnetic component’ (B1) of the electromagnetic RF pulse. The nuclei gain energy and sway away from the perpendicular to the horizontal (90 degree) depending on how long the RF pulse is applied. So, now the nuclei behave like ‘punch-drunk’( like a person who’s been reeling due to a strong blow to the head!).
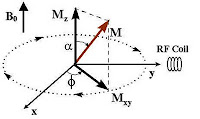
The excited proton recovers in two ways and both forms occur simultaneously: (1) The excited nuclei which now have been ‘forced’ to lie horizontally (90 degree), ‘re-align’ themselves back to their ‘original position’ as they were before the RF pulse (perpendicularly towards the field of externally applied field B0); and (2) the energized protons dissipate their energies to the surrounding nuclei (horizontally) at their level. The first example, obviously, is called the (spin-lattice, or longitudinal) relaxation; while the second one, transverse relaxation (T2). There is little energy loss due to RF emission.
T1 relaxation, also known as, longitudinal relaxation or spin-lattice relaxation can be best understood if you see the following Youtube video. [The spiral trajectory, in this case, reminds me of the laser experiment I did to satisfy my lesser friends. Analogically, the trajectory would be such, if the power supply were switched off.] In T1 relaxation, the proton loses energy to the surrounding lattice, by interacting with nuclei in the lattice which are in vibrational, translational and rotational motion. Clearly, the surrounding nuclei (lattice) having the same (or nearly same) Larmor frequency will efficiently absorb energy of the excited proton, resulting in a tiny rise of temperature.
T2 relaxation (transverse or spin-spin relaxation) on the other hand, does not involve exchange of energy with the lattice.
The magnetic moments of the protons merely changes phase. Here, the nuclei exchange “quantum states” (kind of, what Einstein called ‘spooky action at a distance’): an excited nucleus (proton) will transfer its energy and relax, while the neighboring nucleus in the lower energy state that absorbs it becomes excited. This loss of phase coherence of spins can be clearly seen in this beautiful video.
It can be understood easily that T1 and T2 values would depend on the surrounding molecular environment (tissues, for example). Hence, the values differ in different tissues. Again, since Mxy decays faster, as described, it may be understood why T1 is greater than T2 (usually, T1=5T2). Both T1 and T2 contribute toward contrast in tissues. T1 relaxation time is the time needed for 63% of protons to return to their previous equilibrium state. Likewise, T2 relaxation time is the time needed for 63% of protons to become dephased owing to their interaction with nearby protons. The contrast, naturally depends on the water content of the tissues. Grey matter has about 10% more water than white matter and this creates a contrast. We can also create contrast by varying TR and TE times.
TR (Repetition Time) refers to the time gap at which consecutive RF pulses are applied; while TE (Echo Time) refers to the time delay between the applied RF pulse and its reception (echo). T1 weighted images (T1W) are produced by keeping TR and TE relatively short, while T2 weighted images (T2W) are produced by keeping TR and TE relatively long. Water molecules being relatively light spins much faster than the Larmor frequency, making energy transfer rather tough (exchanging of packets of energy becomes more efficient as the relative angular velocity narrows). Consequently, water has a long T1 time. Proteins and nucleic acids being rather heavy, spin slowly. They also have problem with energy exchanging, and thus have a long T1. Cholesterol, a medium sized molecule, precesses near the Larmor frequency, efficiently absorbing the energy and giving a small T1 value.Thus (fat) liquid cholesterol in craniopharyngiomas, a benign tumor, appears bright on T1W images (T1 being small, the rate at which RF energy is released is fast. Hence, the signal intensity in NMR is high).
Subacute hemorrhage also has shorter T1, due to the presence of paramagnetic iron in methemoglobin present in the tissue, hence high signal intensity. Cerebrospinal fluid (CSF), edema (collection of fluid in tissue space or ECF) having more water content have both long T1 & T2 relaxation time. They give low signal intensity in T1 (dark) but higher signal intensity (bright) in T2W images. T2W images are superior to their T1 counterparts in case of infarction, edema, demyelination etc. Contrast agents like the heavy metal Gadolinium, a paramagnetic substance, has been used to reduce both T1 and T2 times by introducing inhomogeneity in the magnetic field. Gadolinium is complexed (chelated) with a substance called DTPA to prevent toxic build-up inside body tissues. This gives high signal in T1W but a low signal in T2W. It (the complex) does not cross the blood brain barrier (BBB); but disruption in the BBB or parts of the brain where it is deficient (circumventricular organs), take-up the substance and affects relaxation properties.
Below is an MRI showing changes in Subacute Sclerosing Panencephalitis, a complication of measles.

Given all these, it can be said that relaxation parameters of nuclei have enabled us in visualizing biological tissues nonivasively, identifying chemicals spectroscopically and a lot more as we shall see later.
- NMR spectroscopy
- Magnetic Resonance Imaging
- Principles of NMR
- William P. Dillon. Neuroimaging in Neurologic Disorders. In: Harrison's Principles of Internal Medicine, 17th Ed., Volume 2, McGraw Hill; 2008. p. 2491-2497.
- Ian L. Pykett, Ph.D., Jeffrey H. Newhouse, M.D., Ferdinando S. Buonanno, M.D., Thomas J. Brady, M.D., Mark R. Goldman, M.D., J. Philip Kistler, M.D., & Gerald M. Pohost, M.D. (1982). Principles of Nuclear Magnetic Resonance Imaging Radiology
- Time constants
No comments:
Post a Comment